Geothermal Energy
This report was last updated in December 2023. Download the report here, or read the full text below.
Table of contents
Summary What is geothermal energy?
Geothermal energy can be harnessed for carbon-free electricity and heat.
Access to geothermal energy is geology-dependent and varies world-wide.
How could geothermal energy reduce greenhouse gas emissions?
Geothermal energy can help enable a clean energy transition.
Technology improvements can unlock more geothermal energy.
Philanthropic support for next-generation geothermal technologies: theory of change
Examining the assumptions behind our theory of change
What is geothermal energy’s comparative cost profile?
Is there room for more funding?
How does federal funding for the geothermal sector compare to other sectors?
How could philanthropic dollars catalyze additional investment?
Are there major co-benefits or adverse effects?
Key uncertainties and open questions
Summary
What is geothermal energy? Geothermal energy is heat generated deep within the Earth through natural geophysical processes. It can be used for heating and electricity generation. Geothermal power plants can supply consistent baseload power throughout the day, and some new types of geothermal power plants can potentially provide flexible, dispatchable generation and energy storage.
How could geothermal energy reduce greenhouse gas emissions? Geothermal energy can supply carbon-free heat and electricity in place of burning fossil fuels. As a source of clean firm power, geothermal can complement wind and solar power and help diversify clean energy portfolios. We think an energy portfolio that includes geothermal energy provides a more feasible path to net-zero emissions than one based on intermittent renewables alone.
Philanthropic support for geothermal energy - theory of change: We believe that supporting nonprofit organizations focused on next-generation geothermal technologies–such as enhanced geothermal systems (EGS) and advanced geothermal systems (AGS)–could be promising for expanding applications of geothermal energy globally. For example, we think philanthropic support for geothermal innovation could reduce risks for companies, catalyze more funding from traditional financing mechanisms, accelerate learning-by-doing, and drive down costs faster than the counterfactual.
What is geothermal energy’s comparative cost profile? This report does not contain a quantitative cost-effectiveness model, though we hope to release one in the future. Instead, we did a comparative analysis with nuclear advocacy by comparing estimates for the levelized cost of electricity (LCOE) for EGS and small modular reactors (SMRs) in 2050 against each other. We think EGS technologies could become more cost-competitive than SMRs in the future, but given the (1) overlapping cost estimates and (2) our belief that baseload power sources also need to be diversified, especially given that nuclear may work in contexts where geothermal does not and vice versa, we are not strongly convinced that we should persuade donors to support one technology over the other.
Is there room for more funding? Historically, geothermal innovation has received low funding from the private sector because of its high capital costs and risks. Although there has been growing interest in geothermal energy, we think philanthropic support remains additional.
Are there major co-benefits or adverse effects? Co-benefits include geothermal power plants’ smaller land footprint compared to other generating technologies, improved air quality compared to continued fossil fuel usage, and job opportunities for former fossil fuel workers. Adverse effects include risks of contaminated groundwater and induced seismicity for some types of geothermal systems.
Key uncertainties and open questions: There is uncertainty on how quickly the cost of next-generation geothermal technologies will decrease with increased production. We are also concerned about potential conflicts of interest arising from the oil and gas industry's involvement in scaling geothermal.
Bottom line / next steps: We believe increased deployment of next-generation geothermal technologies holds promise in reducing greenhouse gas emissions and requires additional funding. As part of our 2023 research process, we closely examined nonprofit organizations that support geothermal innovation, which could enhance its expansion in the US and abroad. We think the following approaches used by nonprofits are especially promising for expanding geothermal globally: (1) advocating for policies that support geothermal energy research, development, and deployment in the US; (2) de-risking early-stage deployment of next-generation technologies to spur additional investment. Ultimately, we classified Project Innerspace and Clean Air Task Force, both advocates of geothermal technologies, as top recommendations in 2023.
What is geothermal energy?
Geothermal energy can be harnessed for carbon-free electricity and heat.
Geothermal energy, or heat generated within the Earth, can heat reservoirs of water at great depths below the Earth’s surface.[1] Water or steam from these reservoirs can reach the surface via hot springs or geysers, but most hydrothermal reservoirs are locked at high pressure underground.[2] Geothermal power plants generate electricity by drawing very hot water from these reservoirs to the surface, producing steam that drives turbines to generate electricity.[3] Heat extracted from reservoirs and waste heat from geothermal power plants can provide direct heat for buildings and industrial processes.[4] Geothermal energy is a renewable resource because natural geophysical processes continually replenish extracted heat.[5]
Access to geothermal energy is geology-dependent and varies world-wide.
Access to geothermal energy depends on local geology. Characteristics that influence geothermal well productivity include the heat source, rock permeability, and fluid flow patterns underground.[6] Temperature also increases with depth in the Earth.[7] Typically, the most active geothermal resources are located near significant tectonic plate boundaries, such as the Ring of Fire, the region outlining the Pacific Ocean.[8]
Currently, the United States leads as the greatest producer of geothermal electricity, generating 17 billion kWh in 2021 or approximately 0.4% of its overall electricity production.[9] Indonesia follows closely, producing 16 billion kWh in 2021, about 5% of its total electricity production.[10] Among all nations, Kenya has the greatest percentage (43%) of its electricity generated by geothermal power plants.[11]
How could geothermal energy reduce greenhouse gas emissions?
Geothermal energy can help enable a clean energy transition.
Geothermal energy can provide carbon-free electricity and heat without the need for burning fossil fuels. For example, geothermal power plants can replace coal-fired power plants by offering a continuous and reliable source of electricity.[12] Also, certain types of geothermal power plants can store energy and adjust their output, complementing variable renewable energy sources like wind and solar.[13] Geothermal energy can also provide zero-carbon heat for "hard to decarbonize" industrial processes.[14] In general, we think an energy portfolio that includes geothermal energy provides a more feasible path to net-zero emissions than one based on intermittent renewables alone.[15] Additionally, we think research adjacent to geothermal energy, such as gravity batteries and lithium recovery from geothermal brine, could enhance energy storage technologies.[16] However, we have not thoroughly explored those particular research areas.
Technology improvements can unlock more geothermal energy.
Geothermal electricity generation is currently dominated by conventional geothermal, or hydrothermal, systems, which are limited to regions with active volcanism or continental plate boundaries.[17] Research, development, demonstration, and deployment (RDD&D) of next-generation geothermal technologies could tap greater amounts of previously inaccessible geothermal energy. For example, enhanced geothermal systems (EGS) are man-made geothermal reservoirs created by injecting water deep underground and do not require naturally occurring hydrothermal reservoirs (Figure 1). According to the US Department of Energy (DOE), “With technology improvements, EGS could be engineered cost effectively wherever there is hot rock at accessible depths, enabling economic capture of EGS potential nationwide.”[18] Indeed, there have been research efforts to advance EGS such that geothermal heat can be accessed anywhere in the world.[19]
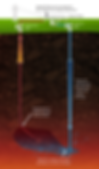
Figure 1: Conceptualization of enhanced geothermal systems (US Department of Energy, "GeoVision" 2019)
Next-generation geothermal technologies are at different stages of development.
The initial focus of EGS RDD&D has been on “in-field” resources within existing conventional geothermal locations (Figure 2). The next stage would likely involve “near-field” resources at the same depth but in less permeable rock.[20] Technology advancements may eventually unlock deep EGS, which offers higher resource potential.[21] Superhot rock geothermal energy, a proposed energy source on the far end of the EGS spectrum, could significantly increase energy extraction and conversion efficiency.[22] According to Altarock Energy, this technology could produce 40-50 MWe of energy per well, compared to 7 MWe per conventional geothermal well and less than 5 MWe per EGS well.[23] Advanced geothermal systems (AGS) are a separate category of closed-loop geothermal wells that recirculate fluid and do not inject water into the ground.[24] We group EGS and AGS technologies together as “next-generation” technologies that are distinct from conventional geothermal technologies.

Figure 2: Geothermal resources and applications, delineated within three resource categories: geothermal heat pump, hydrothermal, and enhanced geothermal systems (US Department of Energy, "GeoVision" 2019)
Next-generation geothermal technologies can increase geothermal’s share of global electricity generation.
According to a 2018 report by the Intergovernmental Panel on Climate Change (IPCC), geothermal energy has the capacity to satisfy approximately 3% of global electricity demand and 5% of heating demand by 2050.[25] In comparison, geothermal power plants accounted for about 0.3% of global electricity generation at the end of 2008.[26] Meanwhile, a DOE report found that technology improvements could increase geothermal power almost 26-fold in the US, reaching 60 GWe of electricity generation by 2050. This would reach 3.7% of total US installed capacity in 2050, and 8.5% of all US electricity generation.[27] We prioritized support for next-generation geothermal technologies over conventional geothermal technologies based on our perception of their difference in scale.
Philanthropic support for next-generation geothermal technologies: theory of change
We think that scaling next-generation geothermal technologies requires an acceptable policy environment, demonstrated technologies, commercial viability, an acceptable regulatory environment, and a feasible implementation pathway (e.g., community acceptance). From what we’ve observed, nonprofits have addressed these barriers by
Advocating for policies supporting geothermal RDD&D in the US, such as increased research funding, optimized permitting timelines, and tax incentives.
De-risking the early stages of next-generation geothermal projects to spur additional investment from traditional financing mechanisms.
Transferring assets from the oil and gas (O&G) sector, including advocacy for reusing O&G wells, technical assistance for replacing O&G power plants with geothermal, and retraining O&G workers.
We evaluated these approaches based on our understanding of their scale, feasibility, and funding need, Table 1. (For more information on these metrics and our research process, see Giving Green’s Research Overview.)
Based on our assessment, we think advocating for geothermal RDD&D policies and de-risking projects to spur private sector investment could be especially promising for supporting next-generation geothermal technologies on the basis of scale, feasibility, and funding need. We explored these approaches further by developing a theory of change (Figure 3) that describes how these approaches could reduce emission and then examined the assumptions behind our theory of change.
Table 1: Scale, feasibility, and funding need of various approaches nonprofits use to help scale next-generation geothermal technologies
Approach | Scale | Feasibility | Funding need | Notes |
Advocating for policies supporting next-generation geothermal RDD&D in the US | High | Medium | Medium | Scale: We think next-generation geothermal has the potential for large-scale deployment by opening up new locations. For example, DOE's GeoVision report said that technological advancements could increase geothermal power production almost 26-fold by 2050, reaching 60 GWe. Feasibility: The political environment seems favorable for policies promoting next-generation geothermal RDD&D, given its recent legislative support (e.g., Inflation Reduction Act, Infrastructure Investment and Jobs Act). However, it is uncertain how quickly new technologies will scale and reduce in cost, as many are not yet proven. Obstacles include high capital costs, high early-stage risks, maintaining public acceptance, and long permitting timelines. Funding need: Geothermal RDD&D receives less federal funding compared to nuclear power, and according to geothermal experts we spoke to, the geothermal industry is less established than the nuclear industry in the US. Although several large nonprofits advocate for geothermal innovation, it is not a dominant focus in their portfolios. |
De-risking projects to spur private sector investment | High | High | Medium | Scale: We rated this as having a high scale because it would support next-generation geothermal technologies. Please see our notes for "Advocating for policies supporting next-generation geothermal RDD&D in the US" for more information. Feasibility: Based on conversations with geothermal experts, we think there are certain products and services that could help the entire geothermal sector but have been neglected by private firms. An example product would be a publicly available global high-resolution map of geothermal resources, which could help companies reduce some early-stage risks related to exploration (e.g., spending money but failing to find geothermal resources that are suitable for commercially viable production). We think this tool seems especially feasible because from our understanding, mapping has been held back by labor and financial constraints. Directly funding first-of-their-kind projects could also de-risk projects and catalyze more investments from traditional financing mechanisms. We rated spurring private sector investment as having high feasibility because we think it is relatively more straightforward than changing policy. Funding need: We found one nonprofit organization that creates public goods and funds first-of-their-kind projects to spur private sector investment. We downgraded this metric to "Medium" because we think companies and governments could fill part of this funding need, especially in wealthier countries. For example, we think DOE’s Geothermal Technologies Office could work with state geological surveys to identify and map geothermal resources in high-priority regions in the US. |
Transferring assets from the O&G sector | Did not assess | Did not assess | Low | Scale and feasibility: In terms of retraining O&G workers, we do not think our methods for assessing scale and feasibility apply well to this pathway because while it seems likely that philanthropy could help nonprofits increase the skilled workforce, we are unsure whether this is currently a primary bottleneck to geothermal deployment. At the same time, we recognize this pathway could have second-order effects that could reduce emissions, such as building political will for geothermal and increasing the likelihood that pro-geothermal policies are passed. As for reusing O&G wells and converting O&G power plants, our impression is that these sites are not necessarily co-located with attractive geothermal gradients and probably not low-hanging fruit for accelerating learning-by-doing. We think other efforts like policy advocacy and spurring private sector investment could have a bigger impact on learning-by-doing and driving down costs, although we are uncertain about this. We did not assess the scale and feasibility of this pathway in depth because we deprioritized this on the basis of funding need. Funding need: We are under the impression that this pathway could have a low funding need because it already has substantial support. According to geothermal experts we spoke to, most US O&G companies have geothermal teams and are thinking about a workforce transition. Within the US, this pathway has also received federal funding, including a 10-year $165 million DOE grant supporting technology transfer and workforce adoption. |

Figure 3: The theory of change behind philanthropic support for next-generation geothermal technologies
In our theory of change, we think policy advocacy and spurring private sector investment could help reduce financial risks and enable technology improvements, leading to reduced costs and faster learning compared to the counterfactual. We think these outputs would decrease emissions if expanded applications of geothermal energy reduce reliance on burning fossil fuels and help speed up and enable a clean energy transition. We also think that if companies can successfully expand next-generation geothermal technologies and replace fossil fuels, this could lead to a positive feedback cycle where the likelihood of supportive policies increases, driving further technology improvements. We explore reasons why we think our assessment of nonprofit strategies and theory of change could be wrong in the section “Key uncertainties and open questions.”
Examining the assumptions behind our theory of change
Below, we discuss and evaluate key assumptions related to our theory of change. For each of the assumptions, we rank whether we have low, medium, or high certainty about the assumption.[28] Our assessment is based on both primary and secondary evidence, as well as our general impression of the plausibility of the assumption. Importantly, a number of the stages of geothermal power’s theory of change may not be amenable to easy measurement or quantification, are not supported by a robust evidence base, or are expected to occur in the future but have not occurred as of yet.
1. The current policy environment is acceptable for enhancing geothermal power RDD&D in the US (high certainty)
We have high certainty that there is currently a favorable policy environment for passing legislation and approving budget requests that would enhance geothermal power RDD&D in the United States. Our impression is based on recent government support for geothermal RDD&D, which we think could be predictive of continued support. For instance, the 2020 Energy Act marked the first reauthorization of DOE’s Geothermal Technologies Office in over a decade, allocating $170 million (USD) annually from FY 2021 to FY 2025.[29] More recently, the bipartisan Infrastructure Investment and Jobs Act (IIJA) included $84 million to support pilot demonstration sites for EGS, and the Inflation Reduction Act (IRA) extended tax credits for renewables, including geothermal power.[30] Additionally, DOE has set a goal to reduce the cost of EGS by 90% by 2035, which we saw as a strong signal of support.[31] Also, our impression is that geothermal power has bipartisan appeal and there has been a history of bipartisan support for geothermal incentives, which we think improves the odds that supportive policies will be passed in a divided Congress.[32]
2. The regulatory environment in the US is acceptable for geothermal innovation (medium certainty)
We have medium certainty that the regulatory environment in the US is acceptable for geothermal innovation. Generally, we believe that the timelines for permitting geothermal projects on federal land could be improved, but that there are potential options for expediting the process and alternatives such as building on private or state-owned land.
The duration required for designing, permitting, approving, and building a geothermal project directly impacts the project’s cost and early-stage risks. Hence, we believe that project timelines strongly influence the extent to which next-generation geothermal projects can be expanded in the US. Currently, geothermal projects that are sited on federally-managed land may have to undergo the environmental review process as many as six times.[33] This additional delay can extend the permitting period from 1-3 years to 5-7 years.[34] Our understanding is that this timeline exceeds the window for applicable tax incentives from the IRA, undermining its effectiveness in expanding geothermal in the US. Considering that 67% of geothermal electricity generation capacity in the US. is located on public land overseen by the Bureau of Land Management, we consider permitting timelines a significant hurdle to expanding geothermal power.[35]
Nevertheless, we are cautiously optimistic that permitting timelines will improve in the future. For example, there is potential to streamline geothermal project permits by calling for categorical exclusions, which are already in place for certain O&G and hydroelectric projects.[36] Various large nonprofit organizations across the political spectrum have advocated for this change, suggesting the potential for bipartisan support.[37] It is also worth noting that opportunities to advance geothermal power extend beyond federal lands, such as in Texas, where most of the state’s geothermal resources are located on private or state-owned land.[38]
3. Geothermal innovation in the US will increase the likelihood of global adoption (medium certainty)
We have medium certainty that geothermal innovation in the US will increase the likelihood of global adoption. Our impression is that RDD&D efforts in the US include technologies that can expand geothermal power globally, and not just domestically.[39] Our impression is supported by a report by Boston Consulting Group and Third Way, which said that the US could leverage its early lead in intellectual property and its O&G workforce to export expertise in drilling, exploration, and innovation.[40] As a caveat, we think geothermal energy's site-specific nature may hinder its export potential. In particular, we are under the impression that variations in drilling technologies and techniques across locations, driven by differences in heat depth and rock hardness, could limit knowledge sharing and affect the rate at which costs go down with increased production.
Nonetheless, we believe certain research activities can support geothermal deployment globally.[41] For example, before drilling and testing, there is considerable uncertainty regarding the quality of geothermal resources at a given site and developers can fail to find resources suitable for commercially viable production. We think geothermal resource mapping can enhance the likelihood of project success by helping with mitigating risks, shortening development timelines (and therefore reducing costs), and attracting more investors.
4. The geothermal sector has sufficient trust and legitimacy to operate (medium certainty)
We have medium certainty that the geothermal sector has sufficient trust and legitimacy, or a social license to operate. For the U.S., our impression is partly informed by a 2022 YouGov poll of US adult citizens, that indicated net favorability (higher likelihood of positive perception compared to negative) of geothermal power among both Democrats and Republicans.[42] However, we think a positive overall impression of geothermal power will not necessarily prevent local opposition. For example, a proposed geothermal project in Nevada has been opposed by community members who expressed concerns about potential subsidence and impacts on their drinking water.[43] Our discussions with geothermal experts suggest that awareness of the geothermal sector remains low in the US and we think that concerns around geothermal’s potential adverse effects could hinder expansion.[44] Various studies in countries including Italy, Australia, Indonesia, Japan, and Chile have also indicated low public understanding of geothermal energy technologies.[45] We think public support for geothermal power will require continued advocacy, education, and outreach.
5. Geothermal innovation will decrease the cost of accessing geothermal energy and increase its geographic range such that companies can increase production globally (high certainty)
We have high certainty that geothermal innovation will decrease the cost of accessing geothermal energy and increase its geographic range such that companies can increase production globally. We discuss our uncertainty about geothermal’s future cost-competitiveness in the section “Key uncertainties and open questions.”
Conventional geothermal power plants have high capital costs, but low maintenance costs, making them cost-competitive over time.[46] It’s unclear what the levelized cost of electricity (LCOE) of next-generation geothermal systems will be because they are still under development.[47] According to the IPCC, EGS will probably cost more than conventional geothermal systems, but EGS will probably become cheaper as its technology improves over time.[48] In fact, one of DOE’s goals is to decrease EGS costs by 90% to $45 per MWh by 2035.[49] If this works, electricity from EGS could be more cost-competitive than electricity from advanced nuclear power, which could have an LCOE of $85 per MWh in 2040.[50]
Although geothermal projects are complex and customized, we think EGS’ price decline is realistic because aspects of EGS could enable learning-by-doing. For example, Tim Latimer (Co-Founder and CEO of Fervo Energy) said EGS can become cheaper similarly to how hydraulic fracturing became cheaper over time.[51] Namely, both technologies can modify rock permeability and create the same wells over and over, enabling learning-by-doing.[52] We also think that EGS will unlock more sites available for geothermal development, which would increase deployment and help drive down costs.
Geothermal’s high capital costs and early-stage exploration costs pose financial risks for companies. Although advancements in geothermal innovation may lower costs and mitigate risks to some extent, we think financial and risk insurance schemes remain necessary.
What is geothermal energy’s comparative cost profile?
In past reports about specific sectors like decarbonizing heavy industry and nuclear, we conducted cost-effectiveness analyses (CEAs) that acted as rough plausibility checks to estimate how cost-effective it could be to support those efforts. This report does not include a CEA for geothermal energy, though we hope to release one in the future. Instead, we provide a comparative analysis with nuclear power, which is another sector we recommend. Although we do not think geothermal and nuclear power are direct substitutes for one another, we thought a comparison between the two would be apt because they can play similar roles in the grid.[53]
We explored LCOE projections from the National Renewable Energy Laboratory (NREL) to compare several types of EGS power plants against small modular reactors, which can potentially be built more quickly and cheaply than traditional nuclear reactors (Figure 4).[54] NREL explored three potential scenarios for EGS that varied in how quickly the technology progresses. The conservative case assumes that current industry trends in drilling and EGS will lead to minor decreases in capital expenditures by 2035.[55] The moderate case assumes that cost improvements are fully achieved across the geothermal industry by 2035.[56] The advanced case assumes substantial advancements in technology by 2035 and streamlined permitting.[57] We compared these cases against LCOE data for small modular reactors (SMRs), which included a moderate and conservative case.[58]

Figure 4: LCOE of near-field and deep EGS under the (a) conservative, (b) moderate, and (c) advanced scenarios. The conservative and moderate cases include a comparison against SMRs. Data: NREL 2023.
In the advanced scenario, EGS’ LCOE in 2050 ranges between $39 and $67 per MWh, depending on the specific technology (Figure 4c). For the moderate scenario, the range is $85 to $137 per MWh, and for the conservative scenario, the range is $127 to $294 per MWh (Figure 4b). In comparison, SMRs reach an LCOE in 2050 of $74 per MWh in the moderate scenario and $88 per MWh in the conservative scenario (Figure 4a). Based on NREL’s analysis, we think the drilling advancements in the moderate scenario are likely achievable and that the conservative scenario for EGS may be especially pessimistic.[59] We also think ongoing goals at DOE, NREL, and other research facilities could push EGS into the advanced scenario and that this could become more attainable if the geothermal sector receives more support, although we are uncertain of this. We think EGS technologies could become more cost-competitive than SMRs in the future, but given the (1) overlapping cost estimates and (2) our belief that power sources need to be diversified, especially given that nuclear may work in contexts where geothermal does not and vice versa, we are not strongly convinced that we should persuade donors to support one technology over the other.
Is there room for more funding?
We think funding to scale up applications of geothermal energy is currently insufficient, and nonprofit organizations supporting geothermal RDD&D would probably benefit from additional contributions.
How does federal funding for the geothermal sector compare to other sectors?
Our impression is that geothermal RDD&D in the US is underfunded compared to RDD&D for other renewables. In 2023, DOE allocated $318 million to solar RDD&D, $132 million to wind RDD&D, and $118 million to geothermal RD&D.[60] However, it is important to note that comparing spending on geothermal power to solar and wind power has its limits. Namely, we see geothermal power as more of a complement to wind and solar than a substitute. We think a more apt comparison may be between geothermal and nuclear power, which can both provide baseload power.[61] Therefore, it may be more relevant to compare RDD&D spending on geothermal and nuclear power relative to their potential to power the US electrical grid. Based on rough estimation using the International Energy Agency’s Net Zero by 2050 model and current US RDD&D spending, we think funding for geothermal power lags behind that of nuclear power, although we are uncertain of this.[62]
How could philanthropic dollars catalyze additional investment?
We think interest in geothermal energy has increased in recent years. Looking at ten representative geothermal start-up companies, venture capital and private equity investments rose from $41 million in 2019 to $242 million in 2022.[63] Also, the President’s Office has requested that funding for DOE’s Geothermal Technologies Office nearly double between 2023 and 2024.[64] Although we think this increased budget request is unlikely to materialize, we think it serves as a strong indicator of interest.[65]
We also think philanthropic donations to geothermal RDD&D have likely increased in recent years, as indicated by the recent entry of major funders such as Schmidt Futures and the Grantham Foundation for the Protection of the Environment into the field.[66] We are unsure of how many philanthropic dollars have gone towards supporting geothermal, but note that the number of funders seems relatively small compared to support for other clean energy sources.
Despite the growing interest, we think there are still opportunities left unfunded in the geothermal sector. For example, a funder we spoke to explained that federal agencies often prioritize projects that are already receiving funding, leaving the potential for new initiatives unexplored. Moreover, government spending processes tend to be slow, whereas philanthropic dollars can be deployed more quickly. Additionally, we think the private sector does not have a financial incentive to create some public goods that could help reduce project costs and risks for all players. Therefore, we think philanthropy could be especially well-suited for filling that gap and catalyzing additional investment.
Are there major co-benefits or adverse effects?
Next-generation geothermal technologies offer co-benefits like reduced land use compared to other sources of electricity production, job opportunities for former O&G workers, improved energy access in remote areas, and energy independence. Adverse effects include gas and liquid emissions and the risk of triggering local hazards. We detail these co-benefits and adverse effects below.
Co-benefits
Small footprint: Geothermal power plants are compact and use less land compared to other electricity sources. Their footprint is significantly lower than that of natural gas, coal, and ground-mounted solar panels.[67] This reduced land requirement has implications for energy planning, aesthetics, and environmental impact considerations.[68]
Job opportunities: Technical skills used in the O&G industry, such as drilling and reservoir mapping, can be transferred and applied to geothermal projects.[69] We think scaling up geothermal power could offer job opportunities to former O&G workers with minimal additional training requirements, which would contribute to a just transition to clean energy.[70]
Poverty alleviation and energy access in rural areas: Geothermal development could help alleviate rural poverty, especially in remote mountainous areas.[71] Small-scale distributed generation from geothermal power can also improve energy access in remote areas.[72]
Energy independence: Geothermal power can help countries build energy independence because it does not rely on imported fuels.[73] However, expanding geothermal energy will likely rely on imported technical capabilities.
Adverse effects
Gas and liquid emissions: Geothermal fluids may contain mercury, arsenic, radon, and boron.[74] Hazardous chemicals from geothermal power plants, such as arsenic and boron, can be harmful to ecosystems.[75] Geothermal fluids can also contain gases such as hydrogen sulfide, hydrogen, methane, ammonia, and nitrogen.[76] Of these gases, hydrogen sulfide is toxic, but its presence in geothermal fluids is often too low to be considered harmful.[77] Some countries, such as parts of the US and Italy, remove hydrogen sulfide from geothermal power plants while others monitor its levels.[78] Groundwater contamination can be prevented through some engineering practices that are typically mandated by environmental regulations.[79] We think closed-loop geothermal systems probably pose a lower risk of groundwater contamination compared to other geothermal technologies because no fluids exit the system, although leaks could still occur.
Triggering local hazards: Deep drilling projects can impact how frequently micro-earthquakes, hydrothermal steam eruptions, and ground subsidence occur.[80] In 2017, an EGS-type power plant triggered a magnitude-5.4 earthquake in South Korea that injured 135 people and caused about $290 million in damage.[81] According to the IPCC, geological risk assessments may help avoid or mitigate local hazards.[82] There is ongoing research into understanding and mitigating induced earthquake hazards.[83]
Water usage: We do not consider water usage a negative impact of geothermal systems because they typically use non-potable water (e.g. brines) and can be designed to use less water, thereby avoiding competition with sources of drinking water.[84]
Key uncertainties and open questions
How supporting geothermal RDD&D compares to other opportunities that could also help scale applications of geothermal energy: We did not explore some pathways that could help support the geothermal sector because we did not know of nonprofits working on them. For example, we did not look into opportunities that focus on building community support for geothermal and maintaining its social license to operate. We are also unsure how supporting innovation in the US compares to other efforts that are more focused on global knowledge spillover, such as increased collaboration and resource-sharing.
Whether next-generation technologies will advance quickly enough to allow substantially more geothermal power: There is uncertainty on geothermal’s future cost-competitiveness because it is unclear how quickly next-generation technologies will improve and decrease in price. We also think there is some uncertainty on how easy it is to apply skills and techniques from the O&G industry to next-generation geothermal technologies. Ultimately, we think there is still value in supporting geothermal technologies because it diversifies the clean energy portfolio and hedges against the risk of a clean energy transition failing.
Conflicting interests from O&G involvement: Although we see major benefits in O&G involvement in geothermal development, like technology transfer and workforce development, we think there may be conflicting interests if geothermal development is centralized within O&G. For example, we think it is possible that O&G companies may prioritize their existing fossil fuel operations over geothermal energy, which could slow down the transition to cleaner and renewable energy sources. We note that similar concerns have been raised with respect to O&G involvement in direct air capture technologies.[85] We are also concerned that if geothermal development is centralized within O&G, this could potentially stifle competition from smaller players and limit the overall growth of the geothermal sector. We would be less concerned about conflicting interests if geothermal is supported as a separate industry instead of centralized within O&G.
Potential geographic bias: Our view of next-generation geothermal innovation as the most important pathway might be influenced by us primarily speaking to people in the U.S., a hub of innovation. We tried to address our potential geographic bias by speaking to people outside the U.S., including academics and nonprofit leaders, but our network remains U.S.-centric.
Bottom line / next steps
We believe scaling applications of geothermal energy holds promise in reducing greenhouse gas emissions and requires additional funding. As part of our 2023 research process, we closely examined organizations that are focused on expanding next-generation geothermal technologies in the US and abroad. In particular, we think policy advocacy for RDD&D in the US and de-risking geothermal projects to spur additional funding are especially promising. Ultimately, we classified Project Innerspace and Clean Air Task Force (CATF) as top recommendations for reducing climate change. We think Project Innerspace’s strategy of fast iteration and quickly getting next-generation technologies on a learning curve complements that of Clean Air Task Force, whose geothermal workstream focuses on superhot rock geothermal energy, which is further away from technological readiness compared to other EGS types but could offer cheaper and abundant carbon-free energy if it becomes commercially viable. We think this portfolio approach of supporting different geothermal technologies and strategies helps increase the likelihood of advancing the geothermal sector. For more information on our recommendations, see our Project Innerspace and CATF deep dive reports.
Acknowledgements
This work has greatly benefited from the feedback provided by various advisors, experts, and reviewers throughout the research process. Giving Green is grateful to those who shared their time, experience, and ideas. We would especially like to acknowledge the principal reviewer, Arthur Reis, P.G., for providing a deep review of this report during its final stages of development.
Endnotes
Note: This is a non-partisan analysis (study or research) and is provided for educational purposes.
1. “Geothermal energy is heat energy from the earth—Geo (earth) + thermal (heat). Geothermal resources are reservoirs of hot water that exist or are human made at varying temperatures and depths below the Earth's surface.” https://www.energy.gov/eere/geothermal/geothermal-basics
2. “Geothermal water or steam may emanate naturally from the reservoir and manifest at the surface as hot springs or geysers; but most stays trapped underground in rock, under pressure and accessible only through drilling.” https://www.energy.gov/eere/geothermal/articles/geovision-chapter-2
3. “Geothermal power plants draw fluids from underground reservoirs to the surface to produce steam. This steam then drives turbines that generate electricity.” https://www.energy.gov/eere/geothermal/electricity-generation
4. “Aside from electricity production, several of these concepts may also be used for Direct Use heat applications, meaning utilization of produced heat directly to heat buildings, or for commercial applications that utilize heat, like agriculture or industrial processes.” The Future of Geothermal in Texas - Chapter 1: Geothermal and Electricity Production, 2023
5. “Geothermal energy is classified as a renewable resource (see Chapter 1) because the tapped heat from an active reservoir is continuously restored by natural heat production, conduction and convection from surrounding hotter regions, and the extracted geothermal fluids are replenished by natural recharge and by injection of the depleted (cooled) fluids. Geothermal fields are typically operated at production rates that cause local declines in pressure and/or in temperature within the reservoir over the economic lifetime of the installed facilities. These cooler and lower-pressure zones are subsequently recharged from surrounding regions when extraction ceases.” Intergovernmental Panel on Climate Change, "Chapter 4: Geothermal Energy" 2018.
6. “The characteristics of productive geothermal wells reflect a dynamic interplay between three major factors, including: heat source(s) and heat flow in the crust; permeability of faults, fracture networks and geological formations; and fluid flow patterns (such as large-scale groundwater convection, recharge and fluid chemistry).” https://www.nature.com/articles/s43017-021-00154-y
7. “Temperature increases with depth within the Earth at an average of about 25ºC/km. So if the average surface temperature is 20ºC, the temperature at 3 km is only 95ºC. Although direct use applications of geothermal energy can use temperatures as low as about 35ºC, the minimum temperature suitable for electrical generation is about 135ºC. Geothermal resources occur in areas of higher-than-average subsurface temperatures.” https://www.energy.gov/eere/geothermal/articles/handbook-best-practices-geothermal-drilling
8. “The most active geothermal resources are usually found along major tectonic plate boundaries where most volcanoes are located. One of the most active geothermal areas in the world is called the Ring of Fire, which encircles the Pacific Ocean.” https://www.eia.gov/energyexplained/geothermal/where-geothermal-energy-is-found.php
9. “The United States leads the world in geothermal electricity generation. In 2022, there were geothermal power plants in seven states, which produced about 17 billion kilowatt-hours (kWh) (or 17,002,000 megawatt-hours). This was equal to about 0.4% of total U.S. utility-scale electricity generation. Utility-scale power plants have at least 1,000 kilowatts (or 1 megawatt) of electricity generation capacity.” https://www.eia.gov/energyexplained/geothermal/use-of-geothermal-energy.php. Indonesia and Kenya: “In 2021, 27 countries, including the United States, generated about 92 billion kWh of electricity from geothermal energy. Indonesia was the second-highest geothermal electricity producer after the United States—about 16 billion kWh—and equal to 5% of Indonesia’s total electricity generation. Kenya was the eighth-highest geothermal electricity producer at about 5 billion kWh. This was equal to about 43% of Kenya's annual electricity generation, which was the largest percentage share among all countries with geothermal power plants.” https://www.eia.gov/energyexplained/geothermal/use-of-geothermal-energy.php.
10. “Indonesia was the second-highest geothermal electricity producer after the United States—about 16 billion kWh—and equal to 5% of Indonesia’s total electricity generation.” https://www.eia.gov/energyexplained/geothermal/use-of-geothermal-energy.php.
11. “Kenya was the eighth-highest geothermal electricity producer at about 5 billion kWh. This was equal to about 43% of Kenya's annual electricity generation, which was the largest percentage share among all countries with geothermal power plants.” https://www.eia.gov/energyexplained/geothermal/use-of-geothermal-energy.php.
12. “Geothermal power plants produce electricity consistently and can run essentially 24 hours per day/7 days per week, regardless of weather conditions.” https://www.energy.gov/eere/geothermal/geothermal-basics
13. “With near-zero variable costs, geothermal plants have traditionally been envisioned as providing “baseload” power, generating at their maximum rated output at all times. However, as variable renewable energy sources (VREs) see greater deployment in energy markets, baseload power is becoming increasingly less competitive relative to flexible, dispatchable generation and energy storage. Herein we conduct an analysis of the potential for future geothermal plants to provide both of these services, taking advantage of the natural properties of confined, engineered geothermal reservoirs to store energy in the form of accumulated, pressurized geofluid and provide flexible load-following generation. We develop a linear optimization model based on multi-physics reservoir simulations that captures the transient pressure and flow behaviors within a confined, engineered geothermal reservoir.” https://www.sciencedirect.com/science/article/pii/S0306261922002537
14. “Instead of using combustion to convert chemical energy in a zero-carbon fuel to heat, zero-carbon heat can also be harvested directly from environmental sources, like solar radiation and geothermal energy.” https://www.sciencedirect.com/science/article/pii/S2542435120305754
15. We think diverse energy portfolios can protect against disruptions in the market and that it is sensible to invest in a wide range of clean technologies because they each have their own set of advantages and disadvantages.
16. Gravity batteries: “One NREL project, Repurposing Infrastructure for Gravity Storage using Underground Potential energy (RIGS UP), is exploring the commercial viability of gravity-based mechanical storage systems using oil and gas wellbores. The ARPA-E-funded project will store electrical energy as potential energy by lifting a multi-ton weight within a wellbore. Once proven, the technology could also be used inside of inactive geothermal wells for long-term mechanical storage.” https://www.nrel.gov/news/features/2023/full-steam-ahead-unearthing-the-power-of-geothermal.html. Lithium recovery: “Only 1% of lithium used in the United States currently comes from domestic sources. An NREL analysis focused on lithium found that it is economically feasible for geothermal brines to yield approximately 24,000 metric tons of lithium per year, enough to establish a secure, domestic supply of the scarce mineral.” https://www.nrel.gov/news/features/2023/full-steam-ahead-unearthing-the-power-of-geothermal.html
17. Electricity: “CHS comprises nearly all geothermal electrical power generation existing today.” University of Texas, Austin, "The Future of Geothermal in Texas - Chapter 1: Geothermal and Electricity Production" 2023. Extent of CHS: ““While the technology is mature, it is limited in supply globally as locations with sufficient heat and fluid flows for power generation are largely confined to areas with active basaltic volcanism, or continental plate boundaries.” University of Texas, Austin, "The Future of Geothermal in Texas - Chapter 1: Geothermal and Electricity Production" 2023.
18. https://www.energy.gov/eere/geothermal/articles/geovision-chapter-2
19. “NREL is advancing research to access geothermal heat anywhere in the world—even where fractures and fluid do not naturally exist.” National Renewable Energy Laboratory, “Geothermal Anywhere” n.d.
20. Technology progression: “. Developing EGS and deploying EGS-enabling technologies is expected to happen in stages along this resource spectrum. The GeoVision analysis assumes the progression described in this section: from in-field to near-field to deep-EGS deployment.” US Department of Energy, "GeoVision" 2019. In-field resources: “Initial EGS resource development and EGS technology deployment will likely occur with in-field resources, at the sites of existing conventional hydrothermal projects.” US Department of Energy, "GeoVision" 2019. Near-field resources: “Once improved technologies enable the industry to consistently and reliably capture in-field EGS resources, the next likely stage for EGS development would be in the near-field environment, or the zones of hot rock extending beyond the margins of conventional geothermal resources. The areas around existing hydrothermal systems are typically hot as a result of the nearby thermal anomaly and are relatively well characterized, but lack permeability and a connected fracture network.” US Department of Energy, "GeoVision" 2019
21. See Figure 2.
22. “At extremely high heat, the performance of geothermal doesn’t just rise, it takes a leap. When water exceeds 373°C and 220 bars of pressure, it becomes “supercritical,” a new phase that is neither liquid nor gas… For our purposes, there are two important things about supercritical water. First, its enthalpy is much higher than water or steam, meaning it holds anywhere from 4 to 10 times more energy per unit mass. And second, it is so hot that it almost doubles the Carnot efficiency of its conversion to electricity.” Vox, "Geothermal energy is poised for a big breakout" 2020.
24. “Advanced Geothermal Systems (AGS) generate heat and/or electric power through a closed-loop circuit, after a working fluid, such as water or CO2, extracts thermal energy from rock formations at great depths via conductive heat transfer from the geologic formation to the working fluid in the closed loop through an impermeable zone, such as a pipe wall.” Malek et al. 2021.
25. “Considering its technical potential and possible deployment, geothermal energy could meet roughly 3% of global electricity demand by 2050, and also has the potential to provide roughly 5% of the global demand for heating and cooling by 2050.” Intergovernmental Panel on Climate Change, "Chapter 4: Geothermal Energy" 2018.
26. “At the end of 2008, geothermal electricity contributed only about 0.3% of the total worldwide electric generation.” Intergovernmental Panel on Climate Change, "Chapter 4: Geothermal Energy" 2018. GG note: We found more recent articles that also mentioned 0.3% of worldwide electric generation, but could not track down where they found that number and how recent that figure is. “That’s difficult and expensive, which is why geothermal power—sometimes called the forgotten renewable—makes up only about 0.3% of electricity generation worldwide.” MIT Technology Review, "What it will take to unleash the potential of geothermal power" 2021.
27. “Technology improvements could reduce costs and increase geothermal electric power deployment. Improving the tools, technologies, and methodologies used to explore, discover, access, and manage geothermal resources would reduce costs and risks associated with geothermal developments. These reductions could increase geothermal power generation nearly 26-fold from today, representing 60 gigawatts-electric (GWe) of always-on, flexible electricity-generation capacity by 2050. This capacity makes up 3.7% of total U.S. installed capacity in 2050, and it generates 8.5% of all U.S. electricity generation.” US Department of Energy, "GeoVision" 2019
28. We describe our certainty as low/medium/high to increase readability and avoid false precision. Since these terms can be interpreted differently, we use rough heuristics to define them as percentage likelihoods the assumption is, on average, correct. Low = 0-60%, medium = 70-80%, high = 80-100%.
29. “The Energy Act of 2020 provides the first reauthorization of DOE’s Geothermal Technologies program in more than a decade. The law directs DOE to support the development of up to three Frontier Observatory for Research in Geothermal Energy (FORGE) sites to study EGS; requires DOE to demonstrate four EGS projects at potentially commercially viable locations across the nation; establishes a research program on geothermal heat pumps; directs the U.S. Geological Survey to update its geothermal resource assessments; and establishes a program that to advance geothermal computing and reservoir modeling. The Act authorizes $170 million annually for FY 2021 through FY 2025.” Information Technology & Innovation Foundation, "Federal Energy RD&D: Geothermal Technologies" 2021.
30. Infrastructure Investment and Jobs Act: “The U.S. Department of Energy (DOE) today issued a request for information (RFI) to support $84 million in enhanced geothermal systems (EGS) pilot demonstration projects included in President Biden’s Bipartisan Infrastructure Law. The legislation authorizes DOE to support four competitively selected pilot projects that demonstrate EGS in different types of geology.” US Department of Energy, "DOE Launches $84 Million Program to Demonstrate Enhanced Geothermal Energy Systems" 2022. Tax credits: “Eligible for ITC or PTC: multiple solar and wind technologies, municipal solid waste, geothermal (electric), and tidal… Through at least 2025, the Inflation Reduction Act extends the Investment Tax Credit (ITC) of 30% and Production Tax Credit (PTC) of $0.0275/kWh (2023 value), as long as projects meet prevailing wage & apprenticeship requirements for projects over 1 MW AC.” US Environmental Protection Agency, "Summary of Inflation Reduction Act provisions related to renewable energy" n.d.
31. “The Enhanced Geothermal Shot™ is a department-wide effort to dramatically reduce the cost of EGS—by 90%, to $45 per megawatt hour by 2035.” US Department of Energy, "Enhanced Geothermal Shot" n.d.
32. Bipartisan appeal: ““Our challenge is not that we have any enemies,” says Latimer. “If you want to talk to Democrats, we produce carbon-free electricity 24/7 — the last piece of the puzzle for a fully decarbonized electricity sector. If you talk to Republicans, it’s American ingenuity putting our drilling fleet to work on a resource that’s fuel-secure, doesn’t rely on imports, and puts the oil and gas people back to work. It’s a beautiful bipartisan story. The problem is we just don’t get talked about.”” Vox, "Geothermal energy is poised for a big breakout" 2020. Evidence of bipartisan support: The 2021 Bipartisan Infrastructure Law provided funding for EGS projects, and prior to that, there were bipartisan bills supporting geothermal incentives (e.g., Advanced Geothermal Innovation Leadership Act of 2019). Bipartisan Infrastructure Law: “The U.S. Department of Energy (DOE) today issued a request for information (RFI) to support $84 million in enhanced geothermal systems (EGS) pilot demonstration projects included in President Biden’s Bipartisan Infrastructure Law.” Department of Energy, "DOE Launches $84 Million Program to Demonstrate Enhanced Geothermal Energy Systems" 2022 Advanced Geothermal Innovation Leadership Act of 2019: “US Senators Lisa Murkowski (R-Alaska) and Joe Manchin (D-W.Va.) yesterday introduced the bipartisan Advanced Geothermal Innovation Leadership Act of 2019, otherwise known as the ‘AGILE’ Act. This legislation aims to accelerate geothermal energy development in the United States by including provisions for research and development of both existing and enhanced geothermal systems, resource assessment updates, grant program authorization, and improved permitting.” ThinkGeoEnergy, "Bipartisan bill put forward in U.S. to advance geothermal R&D" 2019.
33. “The length and number of environmental reviews for a single geothermal project can impact geothermal deployment (Young et al. 2014). Geothermal projects on federally managed land may be subject to an environmental review process under NEPA as many as six times—from agency land-use planning through construction of a power plant and associated transmission infrastructure (Figure 2-19) (Young et al. 2014).” US Department of Energy, "GeoVision" 2019.
34. “Because additional steps and NEPA analyses are required, confirming the resource is more costly and risky. The delay and need for additional steps can result in a 5–7-year period (rather than a 1–3- year period) for a permit applicant to demonstrate a bankable geothermal development (Beckers et al. 2018, Young et al. 2019).” US Department of Energy, "GeoVision" 2019.
35. Geothermal electricity generation capacity: “Geothermal energy production is an important component of this strategy, with 67 percent of the nation’s geothermal electricity generation capacity coming from BLM-managed public lands.” Bureau of Land Management, "Statement of Michael Nedd" 2022. GG note: This percentage does not consider new geographies that could be unlocked with next-generation geothermal technologies.
36. Potential to streamline permits for some O&G categories: “As discussed in Section 3.2.1.2, the GeoVision analysis included an expansion of categorically excluded activities as one of many pathways for an Improved Regulatory Timeline scenario. A categorical exclusion can be applied when a project’s activities fit within a list of actions that an agency has determined do not significantly affect the quality of the human environment. A categorical exclusion is one option that complies with the National Environmental Policy Act, which is required for projects that are on federal lands, supported with federal funds, or otherwise include a major federal action. Categorical exclusions exist for some oil and gas and geothermal development categories, covering geophysical and exploration activities, including the drilling of temperature gradient holes with no new surface disturbance.” US Department of Energy, "GeoVision" 2019. Examples from licensing for small/low-impact hydroelectric projects: Federal Energy Regulatory Commission, "Small/Low Impact Hydropower Projects" n.d.
37. Organizations that have called for streamlined geothermal permitting include ClearPath, Third Way, and Clean Air Task Force. ClearPath: “Streamline permitting and regulatory requirements to reduce costs and increase installed geothermal capacity.” ClearPath, "Geothermal" n.d.. Third Way: “Streamline barriers to domestic deployment. A favorable permitting and regulatory environment can unlock geothermal deployment, facilitate learning, and help U.S. companies achieve economies of scale.” Third Way, "Geothermal: Policies to help America lead" 2023. Clean Air Task Force: “Barrier 1: Inability to deploy projects. Permitting is a critical bottleneck for many of the stakeholders interviewed. Developing geothermal power facilities requires an extensive permitting process.” Clean Air Task Force, "Policy Brief: Earth Energy Innovation" 2023.
38. “Much of the ‘low hanging fruit’ that is currently technologically enabled for geothermal development in the United States can be categorized as CHS, exists on Federal land, and development of those resources is currently constrained by permitting and regulatory obstacles, not technology challenges (IRENA, 2017). This land ownership obstacle is however, not present in Texas, where a majority of the State’s geothermal resources are found on State or private land, as will be explored in further detail in Chapter 13, State Stakeholders: Implications and Opportunities - General Lands Office and University Lands.” University of Texas, "The Future of Geothermal in Texas" 2023.
39. According to the International Renewable Energy Agency (IRENA), initiatives focused on technologies such as extracting geothermal energy from hot dry rocks, closed loop systems, green hydrogen production, and mineral extraction enable geothermal development globally. Our understanding is that geothermal research in the U.S. is diverse and includes those initiatives. IRENA: "Various innovations and research and development initiatives are ongoing in different countries, with a focus on extraction of geothermal energy from hot dry rock (through an enhanced or engineered geothermal system), large-scale closed-loop systems (which are a type of advanced geothermal system), green hydrogen production, development of supercritical resources and mineral extraction from geothermal brines. Funding these initiatives through grants or equity is expected to enable the development of geothermal globally; improve the efficiency of geothermal electricity generation from lowand medium-temperature resources; reduce resource risks; improve the financial viability of new technologies; and enable the economical extraction of minerals from geothermal brines. Implementing pilot projects will demonstrate technical project viability, as well as boost the confidence of communities, policy makers and other stakeholders." IRENA, "Global Geothermal Market and Technology Assessment" 2023.
40. Export opportunities: “Geothermal also opens up export market opportunities with defendable competitive advantages. Expertise in drilling, exploration, and innovation in new technologies is extremely complex and highly exportable – especially to geopolitically important markets in Southeast Asia, Africa, and Latin America.” Third Way, "Two Paths to US Competitiveness in Clean Technologies" 2023. U.S.’s early advantage: “The US’s early advantage stems from several factors, which include an early lead in intellectual property (IP) and a strong oil and gas (O&G) workforce that can be re-skilled.” Third Way, "Geothermal: Policies to Help America Lead" 2023.
41. “For customized technologies, data related to user preferences and relevant factors in the physical environment (e.g., detailed geological maps for geothermal power, biomass feedstock characteristics and availability for biomass power, data on building stocks for building envelope retrofits) can be considered a public good. Thus, the efforts of such clubs also need to be directed toward collection and dissemination of such data across contexts, which can facilitate aggregation of markets and scaling up of customized technologies.” Malhotra and Schmidt 2020.
42. See Figure: Net favorability of energy sources among Democrats and Republicans. https://today.yougov.com/topics/politics/articles-reports/2023/01/03/how-democrats-and-republicans-view-energy-sources
43. “Loss of drinking water is one of the many concerns Gerlach residents have over Ormat’s proposed project. Another is subsidence, the gradual sinking of land already occurring in certain parts of town.” https://www.nytimes.com/2023/05/17/business/burning-man-geothermal-plant-nevada.html
44. “Awareness and acceptance can influence policies, incentives, land access, and other features crucial to geothermal development. In fact, many barriers to successful renewable projects at the implementation level can be considered manifestations of a lack of social acceptance (Wüstenhagen et al. 2007). For example, the public may not have a clear understanding of EGS projects and/or induced seismicity, which could lead to lower acceptance for future EGS projects.” US Department of Energy, "GeoVision" 2019.
45. Italy: “Our results show that in Termini Imerese there is considerable optimism about geothermal energy exploitation. Nevertheless, levels of uncertainty amongst the general population are high and relates to a substantial lack of knowledge and information on the subject. At the same time, citizens clearly ask for major public participation in energy policy, land management and public fund allocation.” Pellizone et al. 2015. Australia: “A key finding is that the majority of the Australian public report limited the knowledge or understanding of geothermal technology and have various concerns including water usage and seismic activity instigated by geothermal drilling.” Dowd et al. 2011. Indonesia: “Based on the interviews with local communities, they reject the construction of geothermal power plants in Mt. Lawu because they think geothermal power plants will give negative impacts to cultural, environmental, economic, and social life aspects in society. This perception may appear because of the lack of understanding of geothermal energy.” Ibrohim, Prasetyo, and Rekinagara 2019. Japan: “Over 80% of respondents thought that they ‘know’ or ‘know well and can explain in detail’ about each of the various power technologies, including fossil fuel, nuclear, hydropower, solar, and wind power (Figure 7). However, this dropped to 64.2% and 32.5% in the case of respondents’ recognition of geothermal and biomass power, respectively.” Kubota 2015. Chile: “It suggests that there is a low level of understanding of the technology involved in geothermal energy production, and it highlights social barriers such as lack of trust, spiritual relationship to volcanoes, and uncertainty about environmental impact as factors that affect risk and public perception.” Payera 2018.
46. High capital cost and cost-competitiveness: “Over the long-term, geothermal power offers a cost-effective means of achieving aggressive decarbonization pathways; in the short-term, however, developing geothermal systems carries significant up-front costs.” US Department of Energy, Geothermal FAQs n.d. Maintenance cost: “Costs of a geothermal plant are heavily weighted toward early expenses, rather than fuel to keep them running. Exploration activities—pre-drilling geotechnical studies, exploration, confirmation, and development drilling—have a collective impact on overall project costs and success. Most geothermal power plants can run at greater than 90% availability (i.e., producing more than 90% of the time), which means that costs can be recouped more quickly. However, operators need to balance operations with costs and electricity prices. Running at 97% or 98% can increase maintenance costs, but higher-priced electricity justifies running the plant 98% of the time because the resulting higher maintenance costs will be recovered.” US Department of Energy, Geothermal FAQs n.d.
47. LCOE refers to the cost of generating one unit of electricity (measured in megawatt-hours) from particular energy sources over the lifetime of a power plant. It takes into account various costs, including capital costs, operating and maintenance costs, fuel costs (if applicable), and the plant’s expected lifespan.
48. “There are no actual LCOE data for EGS power plants, as EGS plants remain in the demonstration phase, but estimates of EGS costs are higher than those for hydrothermal reservoirs. The cost of geothermal energy from EGS plants is also expected to decrease by 2020 and beyond, assuming improvements in drilling technologies and success in developing well-stimulation technology.” Intergovernmental Panel on Climate Change, "Chapter 4: Geothermal Energy" 2018.
49. “U.S. Secretary Jennifer M. Granholm today announced a new Department of Energy (DOE) goal to make enhanced geothermal systems (EGS) a widespread renewable energy option in the U.S. by cutting its cost by 90% to $45 per megawatt hour by 2035.” See Table B1b. Estimated unweighted levelized cost of electricity (LCOE) and levelized cost of storage (LCOS) for new resources entering service in 2040 (2021 dollars per megawatthour). US Department of Energy, "DOE Launches New Energy Earthshot to Slash the Cost of Geothermal Power" 2022.
50. See Table B1b. Estimated unweighted levelized cost of electricity (LCOE) and levelized cost of storage (LCOS) for new resources entering service in 2040 (2021 dollars per megawatthour). https://www.eia.gov/outlooks/aeo/pdf/electricity_generation.pdf.
51. “Similarly, EGS projects have the potential for far greater scale and repeatability than traditional hydrothermal geothermal projects. Because EGS uses stimulation to induce permeability, places horizontal wellbores to increase reservoir contact, and has the potential to create closed loop systems with water recirculation, the criteria for finding a suitable EGS location are broader than conventional projects. This will allow for large exploitation of resources that have the appropriate thermal gradient and suitable reservoir characteristics that will bring a scale and repeatability similar to unconventional oil and gas development. Because of this, in a similar way that the experience curve is particularly applicable to unconventionals, it is also particularly applicable to EGS development.” Latimer and Meier, 2017.
52. We note that Fervo Energy is an EGS company and is incentivized to advocate for geothermal energy.
53. As a caveat, cost is not the only factor that should be considered when choosing between nuclear and geothermal. Wase, land area, and social license to operate are also important factors.
54. We compared EGS against small modular reactors because we think that the future of nuclear power likely lies with small modular reactors instead of conventional nuclear reactors.
55. “Conservative Technology Innovation Scenario (Conservative Scenario): Continuation of current industry trends in drilling (e.g., minor efficiency improvements with little to no increase in rate of penetration [ROP]) and EGS (e.g., limited increase in flow rate and stimulation success rate) result in minor CAPEX improvements by 2035.” National Renewable Energy Laboratory, "Geothermal" 2023.
56. “Moderate Technology Innovation Scenario (Moderate Scenario): Drilling advancements (e.g., doubled ROP and bit life and reduced number of casing intervals and associated drilling materials) detailed as part of the GeoVision report (DOE, 2019) and EGS stimulation successes from the EGS Collab project (Kneafsey et al., 2022) and industry demonstration projects (Norbeck et al.2023) result in cost improvements that are fully achieved industry-wide by 2035.” National Renewable Energy Laboratory, "Geothermal" 2023.
57. “Advanced Technology Innovation Scenario (Advanced Scenario): substantial drilling and EGS advancements (e.g., significantly increased ROP, bit life, and EGS stimulation success, limited casing intervals, significantly reduced consumption of drilling materials, and reduced timelines) as modeled in the Technology Improvement scenario of the GeoVision report result in cost improvements that are achieved by 2035. Going by the updates made in the 2022 ATB, Advanced Scenario EGS power plants are assumed to be built with 100 MW of capacity to maximize project efficiency. In addition, permitting timelines reflect anticipated permit streamlining effects of a National Renewable Energy Coordination Office, as enacted through the Energy Act of 2020.” National Renewable Energy Laboratory, "Geothermal" 2023.
58. “Base year and future cost and performance data (see above) are sourced from the U.S. Energy Information Administration's (EIA's) Annual Energy Outlook 2023 (AEO2023) projections” National Renewable Energy Laboratory, "Other Technologies" 2023.
59. “Justification: Cost modeling of drilling improvements along with limited successful field demonstrations and abundant oil and gas experience confirm this level of advancement is achievable (Lowry et al., 2017a); (Lowry et al., 2017b); (Hackett et al., 2020).” National Renewable Energy Laboratory, "Geothermal" 2023.
60. Information Technology & Innovation Foundation, "Energizing Innovation in Fiscal Year 2024" 2023.
61. As a caveat, we do not think these two are necessarily substitutes for each other because there are some contexts where geothermal may be more advantageous than nuclear and vice versa. We also note that hydroelectric is also a major baseload clean power source. However, we did not explore hydroelectricity further because it is especially location-constrained.
62. According to IEA’s model, the global electrical capacity of nuclear power in 2050 will be 812 GW compared to geothermal’s 126 GW. Compared to the $118 million that the U.S. spent on geothermal innovation in 2023, it spent $1.3 billion on nuclear RDD&D in 2023. Dividing these two values, we found that the ratio of investment to projected electrical capacity (millions USD/GW) was about 0.94 for geothermal compared to 1.62 for nuclear. This lower ratio for geothermal compared to nuclear suggests that spending on geothermal power could lag behind that of nuclear power relative to their respective potential, although we are uncertain of this. We think our comparison between geothermal and nuclear investments could be wrong if (1) spending on U.S. nuclear innovation only plays a minor role in increasing nuclear capacity globally and (2) supporting U.S. nuclear innovation is not analogous to supporting U.S. geothermal innovation, which could have more opportunities for international spillover effects. Additionally, a geothermal expert told us that IEA’s model underestimates geothermal power’s potential because it only considers conventional geothermal systems. Electrical capacity: Table A.3: Electricity International Energy Agency, "Net Zero by 2050: A Roadmap for the Global Energy Sector" 2021. Federal spending: DOE Energy RD&D programs summary, FY 2021 enacted through FY 2024 request ($ millions) Information Technology & Innovation Foundation, "Energizing Innovation in Fiscal Year 2024" 2023.
63. For more information, see VC/PE investment in next-generation geothermal companies, 2023-10-10.
64. See Table 2: DOE Energy RD&D programs summary, FY 2021 enacted through FY 2024 request ($millions). Information Technology & Innovation Foundation, "Energizing Innovation in Fiscal Year 2024" 2023.
65. We do not think GTO will receive a nearly doubled budget because it seems likely that nondefense discretionary funding in FY 2024 will remain close to FY 2023 levels. “The bill cuts so-called nondefense discretionary, which includes domestic law enforcement, forest management, scientific research and more — for the 2024 fiscal year. It would limit all discretionary spending to 1 percent growth in 2025, which is effectively a budget cut, because that is projected to be slower than the rate of inflation.” New York Times, "New Details in Debt Limit Deal: Where $136 Billion in Cuts Will Come From" 2023.
66. To the best of our knowledge, Schmidt Futures started funding geothermal in 2022 with its support for the Texas Geothermal Institute, and the Grantham Foundation began funding geothermal within the past few years. Schmidt Futures: ““Geothermal could play a much larger role in generating clean, affordable, abundant, and reliable energy,” said Tom Kalil, Schmidt Futures’ Chief Innovation Officer. “Our support aligns with Schmidt Futures’ mission to bet early on exceptional people making the world better. We hope the private sector, philanthropy and policy-makers will work together to foster geothermal’s potential to increase production of renewable energy and create jobs.”” ThinkGeoEnergy, "Texas Geothermal consortium launched to roadmap capabilities and technology gaps" 2022. Grantham Foundation: The Grantham Foundation has used its venture capital vehicle to support geothermal companies including Fervo Energy and Zanskar. The earliest mentions that we found connecting the Grantham Foundation to Fervo Energy and Zanskar are from August 2022. “Other new investors included Canada Pension Plan Investment Board (CPP Investments), Liberty Energy, Macquarie, Grantham Foundation for the Protection of the Environment, Impact Science Ventures, and Prelude Ventures.” Business Wire, "Fervo Energy Raises $138 Million for 24/7 Carbon-Free Next-Generation Geothermal Energy" 2022. “Zanskar closes US$12 m Series A funding co-led by USV and Lowercarbon, with participation from new and existing investors, including Munich Re Ventures, Safar Partners, Grantham Foundation, and First Star Ventures.” ThinkGeoEnergy, "Zanskar reports closure of $12m Series A funding for geothermal discovery tech" 2022.
67. Table 1. Land use intensity of electricity (LUIE) showing total direct and indirect land use (ha/TWh/y). Lovering et al 2022.
68. “People are concerned about the impacts of land use for energy production for several reasons. The first is the technical question of whether we even have enough land to produce all of our energy from particular sources at all. The second is an aesthetic concern about how much of our landscapes might be taken up by these technologies. The third is the impact of land use on natural habitats and the environment.” Our World in Data, "How does the land use of different electricity sources compare?" 2022.
69. “For example, petroleum and gas engineering skills are highly applicable to geothermal, including seismic interpretation, drilling and completions, reservoir mapping or flow assurance.” International Energy Agency, "World Energy Employment" 2022.
70. This impression is partly informed by an interview with Jamie Beard (Project Innerspace) on the Volts podcast. “So skills transfer and all that? Yes, I mean, almost 100%. It is so synergistic in terms of skill set, transferring from oil and gas to geothermal that we're talking about minimal training certificate level, let's just get you up to speed kind of thing, but otherwise go.” Volts, "What's going on with geothermal?" 2023.
71. “This can alleviate rural poverty in developing countries, particularly in Asia, Central and South America, and Africa, where geothermal resources are often located in remote mountainous areas.” Intergovernmental Panel on Climate Change, "Chapter 4: Geothermal Energy" 2018.
72. “In many developing countries, geothermal energy is also an appropriate energy source for small-scale distributed generation, helping accelerate development through access to energy in remote areas.” Intergovernmental Panel on Climate Change, "Chapter 4: Geothermal Energy" 2018.
73. “When it comes to the nation’s future power needs, geothermal energy checks many critical boxes. A renewable resource, it produces no greenhouse emissions and has a low environmental impact. Geothermal energy can be harnessed without importing fuel, and it produces energy around the clock; it could pave the way to increasing the nation’s energy independence.” Pacific Northwest National Laboratory, "Geothermal Energy: Harvesting the Earth's natural heat" n.d.
74. “Mercury, arsenic, radon and boron may be present.” Intergovernmental Panel on Climate Change, "Chapter 4: Geothermal Energy" 2018.
75. “If present, boron and arsenic are likely to be harmful to ecosystems if released at the surface.” Intergovernmental Panel on Climate Change, "Chapter 4: Geothermal Energy" 2018.
76. “Apart from CO2, geothermal fluids can, depending on the site, contain a variety of other minor gases, such as hydrogen sulphide (H2S), hydrogen (H2), methane (CH4), ammonia (NH3) and nitrogen (N2).” Intergovernmental Panel on Climate Change, "Chapter 4: Geothermal Energy" 2018.
77. “Of the minor gases, H2S is toxic, but rarely of sufficient concentration to be harmful after venting to the atmosphere and dispersal.” Intergovernmental Panel on Climate Change, "Chapter 4: Geothermal Energy" 2018.
78. “Removal of H2S released from geothermal power plants is practised in parts of the U.S.A. and Italy. Elsewhere, H2S monitoring is a standard practice to provide assurance that concentrations after venting and atmospheric dispersal are not harmful.” Intergovernmental Panel on Climate Change, "Chapter 4: Geothermal Energy" 2018.
79. “Shallow groundwater aquifers of potable quality are protected from contamination by injected fluids by using cemented casings, and impermeable linings provide protection from temporary fluid disposal ponds. Such practices are typically mandated by environmental regulations.” Intergovernmental Panel on Climate Change, "Chapter 4: Geothermal Energy" 2018.
80. “Local hazards arising from natural phenomena, such as micro-earthquakes, hydrothermal steam eruptions and ground subsidence may be influenced by the operation of a geothermal field (see also Section 9.3.4.7). As with other (non-geothermal) deep drilling projects, pressure or temperature changes induced by stimulation, production or injection of fluids can lead to geo-mechanical stress changes and these can affect the subsequent rate of occurrence of these phenomena.” Intergovernmental Panel on Climate Change, "Chapter 4: Geothermal Energy" 2018.
81. Magnitude: “A South Korean government panel has concluded that a magnitude-5.4 earthquake that struck the city of Pohang on 15 November 2017 was probably caused by an experimental geothermal power plant.” Zastrow 2019. Damage: “The quake was the nation’s second strongest and its most destructive on modern record — it injured 135 people and caused an estimated 300 billion won (U.S.$290 million) in damage.” Zastrow 2019.
82. “Local hazards arising from natural phenomena, such as micro-earthquakes, hydrothermal steam eruptions and ground subsidence may be influenced by the operation of a geothermal field (see also Section 9.3.4.7). As with other (non-geothermal) deep drilling projects, pressure or temperature changes induced by stimulation, production or injection of fluids can lead to geo-mechanical stress changes and these can affect the subsequent rate of occurrence of these phenomena (Majer et al., 2008). A geological risk assessment may help to avoid or mitigate these hazards.” Intergovernmental Panel on Climate Change, "Chapter 4: Geothermal Energy" 2018.
83. “Collaborative research initiated by the IEA-GIA (Bromley and Mongillo, 2008), the U.S.A. and Australia (International Partnership for Geothermal Technology: IPGT)12 and in Europe (GEISER)13, is aimed at better understanding and mitigating induced seismicity hazards, and providing risk management protocols.” Intergovernmental Panel on Climate Change, "Chapter 4: Geothermal Energy" 2018.
84. “Water is not a limiting factor for geothermal power generation, since geothermal fluids are usually brines (i.e., not competing with other uses). Flash power plants do not consume potable water for cooling and yield condensed water that can, with proper treatment, be used for agricultural and industrial purposes. Binary power plants can minimize their water use with air cooling.” Intergovernmental Panel on Climate Change, "Chapter 4: Geothermal Energy" 2018.
85. “But some oil executives seem to have other plans for the technology, which received a big financial boost from the bipartisan infrastructure law and Inflation Reduction Act. DAC plants use fans, filters, power and piping to suck carbon dioxide from the air and permanently store it deep in the earth. Occidental Petroleum Corp. leader Vicki Hollub has described DAC not as a climate solution but a way to continue producing petroleum.” E&E News, "Oil companies want to remove carbon from the air — using taxpayer dollars" 2023.